Claiming significantly higher power generation capacity than traditional blades, Xenecore aims to scale up its current monocoque, fan-shaped wind blades, made via compression molded carbon fiber/epoxy with I-beam ribs and microsphere structural foam. #Work In Progress #windblades
Drag-based wind energy design. Aiming to translate its expertise in manufacturing monocoque, ribbed components with thermoplastic microspheres, Xenecore is working to develop a fan-shaped, drag-based wind turbine design with potential for high energy capture even in low winds. The Fanturbines would ideally be stacked to capture more wind surface area, like in the above rendering. Photo credit, all images: Xenecore Windmill Power Generation
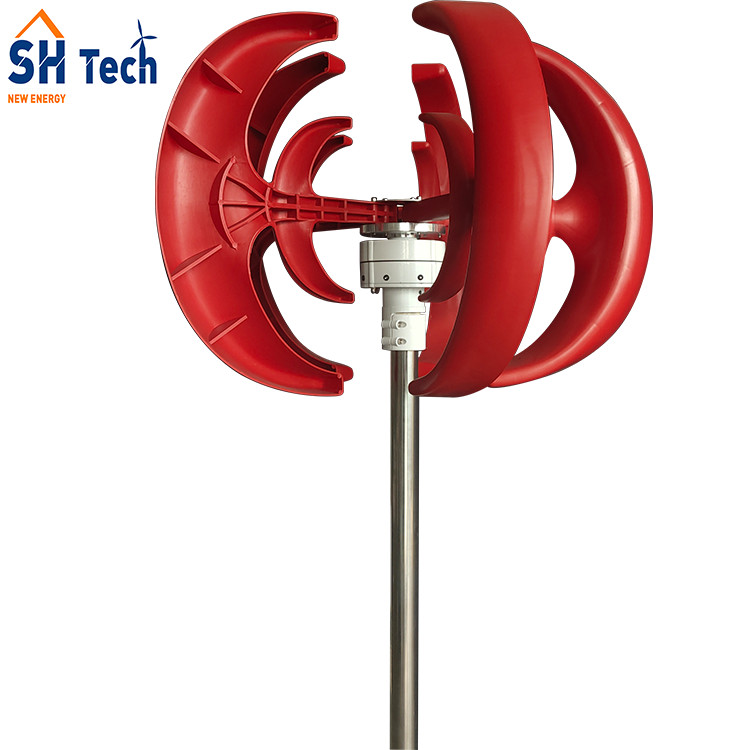
As countries like the U.S. announce policies and set goals focused on growing renewable energy capacity, an opportunity arises to consider new, improved renewable energy technologies. One company aiming to help develop more efficient wind turbine blades with higher energy capture capabilities is Xenecore (New York, N.Y., U.S.), which is leveraging its expertise in thermoplastic microsphere core and compression molded monocoque composite parts into a fan-shaped, drag-based wind blade design.
Founded in 2010, Xenecore got its start with a materials technology for sporting goods applications when CEO and founder Jerry Choe began experiencing tennis elbow from his carbon fiber composite racket. “I unsuccessfully tried every racket under the sun. So, then I decided to develop my own racket, and that’s how it all started,” he says. Choe, a patent attorney, explains that he has a technical background and holds numerous technology patents.
The problem, he says, is that carbon fiber tennis rackets are designed for high performance and power when hitting a tennis ball, but they do not do a good job of mitigating impact to the arm. Typically, these rackets are hollow to minimize weight and are made via a bladder molding process.
Choe set out to find a solution that would more effectively dampen vibrations and their impact upon the arm. After an 18-month development period, he and his team developed a material and process solution that is now marketed as Xenecore, a thermoplastic microsphere product that can be used as a structural core for composite parts.
Composite design using thermoplastic microspheres. To maximize strength for its products, Xenecore combines carbon fiber ribs, or I-beams, with its thermoplastic microsphere film.
Today, Xenecore is available in several formats, including a film sheet for layup within a closed mold, which is how both the company’s tennis racket designs and wind blades are manufactured. The microsphere film expands under heat and pressure to produce a strong structural foam that also serves to dampen vibrations and noise.
“It’s not just adding foam to an existing hollow racket. [Xenecore] is actually part of the structure, and that’s what makes it special,” Choe says. “Our technology is a material and a carbon molding technology.
Following these initial successes, Choe explains that his company has invested heavily into further development of microsphere technology, obtaining more than 250 patents worldwide. The company has also developed a number of industry partnerships including distributor Composites One (Schaumburg, Ill., U.S.), and offers equity in its intellectual property (IP).
In recent years, Xenecore has seen new opportunities to expand the use of its microsphere products beyond tennis rackets into other applications, such as blades for drones and, most recently, a novel design for drag-based wind turbine blades.
About two years ago, Choe and the Xenecore team began investigating ways to leverage the company’s process technology and microsphere product for the development of wind turbine blades. “Combating climate change and promoting renewable energy is high priority, and I knew that our technology could be useful for manufacturing wind blades,” Choe says. He also recognized an opportunity to rethink how these blades are designed.
Most of today’s wind turbines feature long, thin, airplane wing-shaped blades that generate electricity by using lift. When wind passes over the blade, the lower pressure that forms on one side of the blade pulls (in other words, lifts) the blade perpendicular to the direction of the wind, causing it to spin a rotor that transfers energy into the turbine to generate electricity.
These blades are typically made from fiberglass skins, often supported by a carbon fiber composite spar cap in longer blades. Wind blades are generally laid up in open molds, vacuum infused in two halves and then assembled together using shear webs, foam core and adhesive.
The earliest windmills, however, looked very different, featuring wide, flat, fan-shaped wooden blades that generated electricity from drag, where the force of the wind is directly used to push the blade in the direction of the wind. Choe explains, “When wind turbines were first invented, everybody was using drag, because it captures much more wind. But these first blades were a problem because of the materials used.” The very earliest windmills were built from soft, less durable materials like cloth.
In 1919, German physicist Albert Betz published his now-famous Betz’s law, about wind capture and blade design. According to this law, a blade can only capture up to a maximum of 59% of the kinetic energy of wind using lift. This theory informed the way that airplane wings and wind turbine blades are shaped, maximizing lift forces — and minimizing drag — with a thin, curved design that still prevails today.
According to Choe, Betz’s 59% energy capture — which is the maximum only in theory, as actual wind turbines capture energy considerably less efficiently — does not have to be the maximum using today’s materials. The stronger and lighter material options available today, such as fiberglass and carbon fiber composites, easily outperform the metals used to produce wind blades and airplane wings in Betz’s day. “It was the best design at the time, given the materials available, but it’s actually very inefficient, and no longer needs to be the standard,” Choe says.
It’s worth noting that there are a number of drag-based wind turbine blade designs that have long been in use, such as Savonius style vertical wind turbines, which feature two cup-shaped blades that spin around a central turbine. These turbines are generally much less efficient than lift-based turbines, limited by the fact that in a vertical setup, the two blades actually block some of the wind that can be captured by the other half of the blade. However, their simple design and ability to capture energy in low-wind areas has made them popular for use in turbines for home or business environments.
Choe and his team set out to develop an updated horizontal wind turbine that maximizes and uses drag rather than treating it as a loss, as Betz had described — and, notably, using composite materials.
According to a 2021 whitepaper by the late Dr. Paulo Abdala, professor of aeronautics at the University of Brasília (Brazil), one challenge faced by the Xenecore team early on was that because lift-based turbines are the standard, today’s simulation software is designed to analyze the performance of only lift-based turbines. Choe and his team tried a number of physics-based analysis tools and ultimately used Ansys (Canonsburg, Pa., U.S.) Fluent computational fluid dynamics software to model the behavior of wind on the blades.
Nature-inspired design. For its Fanturbine, Xenecore developed ribs to fan outward from the center, inspired by the strength and veins of a palm frond.
Using these models, the goal was to develop a blade that maximizes and captures drag to generate electricity within the turbine, while withstanding high wind loads with as little weight as possible.
The Xenecore team first tried to construct a solid carbon fiber composite blade, “but the weight-to-strength ratio was not good. Even a solid carbon plate will break [from high wind forces],” Choe says.
Ultimately, Xenecore designed a monocoque, fan-shaped blade, called a Fanturbine, comprising top and bottom skins cored with Xenecore thermoplastic microspheres. These skins are reinforced with ribs referred to as I-beams. The design is biomimetic, or nature-inspired, as the ribs fan out from a central point much like the leaves on a palm frond.
The Fanturbine blade is manufactured in a one-step compression molding process, using a very high modulus carbon fiber and epoxy to maximize strength and stability for resisting high wind loads at the lightest possible weight. The one-piece monocoque design also aims to maximize stability as well as to theoretically extend the life of the blade, since there are no seams or adhesives that can be damaged or fatigued over time. Right now, the first versions of these blades are relatively small — up to 3 × 3 feet — with a goal to scale up to larger sizes to compete with traditional wind blades.
“The Xenecore microspheres and I-beams are an ideal solution because they add strength and a bit of thickness, but not much weight,” Choe adds. “The microspheres also help with vibration and noise reduction — there is almost no noise when the turbine is running.”
To produce each blade, cut carbon fiber fabrics for the skins and ribs are laid into aluminum top and bottom molds. Layers of Xenecore film papers are placed on top of each skin. The mold closes, and under heat and pressure, the microspheres expand into a lightweight structural foam that binds to the skins. The process produces a single, seamless, adhesive-free, I-beam-ribbed monocoque part.
Future installation. Today, Fanturbines are sold in small, 5-kilowatt versions for installation on residential or business rooftops (bottom image). In the future, Xenecore hopes to develop larger-scale blades for installation in stacked rows (see opening image) or to work alongside traditional turbines (top image).
Xenecore’s turbine design incorporates four of the fan blades on each turbine, covering about 80% of available surface area. The wind pushes against the blades, rotating the rotor and leading to energy generation in the turbine. According to Abdala’s whitepaper, the amount of power generated is largely dependent on the speed of the wind. The solidity of the flat, fan-shaped blades helps to create a steep pressure difference on either side of the blade, which is said to increase the wind speed and the power generated.
According to Xenecore’s simulations, in theory, the Fanturbine can reach a maximum of 98% of wind energy capture in ideal conditions. Furthermore, the blade is designed to withstand hurricane-level winds — in simulation, it has been shown to withstand up to 376 miles per hour, considerably more than top hurricane speeds.
Since the original publication of this article, Xenecore and a scholar from the University of Strausburg have conducted additional modeling and simulations aiming to further validate the potential energy generation of the Fanturbine, based on 100-meter-long blades in both a 4-blade (1 gigawatt) and 30-blade configuration (3 gigawatts).
For more information, please see Xenecore’s CFD simulations study webpage and report “Computational fluid dynamics simulations on drag-based wind turbine design for higher energy capture.”
Choe says these blades can operate on existing turbines without changing the existing infrastructure. For ease of transportation on the back of a truck, the blades — wider than typical long and narrow wind blades — must be relatively small, but Xenecore envisions that many turbines could be stacked together in a multistory system. “Stacking wind blades like this takes up a much smaller surface footprint than a solar farm,” says Choe, “ which takes up a lot of land.” He adds that these blades also potentially mitigate wildlife concerns, because the wide, flat blades are more visible for flying animals like bats or birds that often get caught in traditional wind blades.”
In 2022, Xenecore began making small, 5-kilowatt turbines with 3 × 3-foot blades, selling to distributors in South America and making them available globally online. These small systems, Choe explains, are designed to replace solar panels of similar wattage used for residences and businesses, providing equivalent power “but they perform much better and at a three times lower [operating] cost.” These blades have been tested to exhibit energy generation of up to seven times that of conventional wind blades of similar size. The largest system Xenecore has tested is a 100-kilowatt turbine with 11-foot-wide blades. It has a megawatt-sized version in design.
Maximizing energy capture. In theory, a large-scale Fanturbine could capture significantly more wind energy than a similar-sized traditional lift-based turbine. Next steps for Xenecore include building and testing prototypes of larger blades.
There is interest in larger Fanturbine blades for the near future, Choe says, noting that this technology has the potential to refit today’s largest Haliade X turbine by GE (Paris, France), which could increase its capacity up to a hundred-fold — from 14 megawatts to 1.4 gigawatts. “That’s what’s possible if you convert from lift to drag energy. That’s what our technology does,” he says, adding that high-energy capture renewable energy solutions like this are needed to meet net-zero targets for combating climate change.
Currently, the company is looking for investors and partners to help launch the technology into the next phase. To prove out the technology, Xenecore next aims to build and install a 1-megawatt turbine onto a retrofitted, decommissioned wind turbine tower.
As longer composite wind blades are developed, customized mesh products help OEMs and kitting companies control resin flow and remove air voids during infusion.
Suppliers, fabricators and OEMs across the composite wind blade supply chain ramp up existing technologies, develop better reclamation methods and design more recyclable wind blades.
Denmark-based Isodan Engineering ApS translated its expertise in mobile, shipping container-based newspaper recycling systems to solve a need for breaking down wind turbine blades on-site for reuse.

Solar Wind Turbine © 2023 Gardner Business Media, Inc. Privacy Policy [Log On]